Foodand Nutrition Sciences Open Access Journals Juniper Publisher
Abstract
Drought stress is a major constraint for crop production in arid and semiarid regions. In this study, plant water status could be considered as one of the most critical criteria not only determining but also controlling the overall plant performance. Water stress greatly decreased root biomass, root density and distribution. On the contrary, root length, number of adventitious roots as well as root/shoot ratio was found to increase as a result of drought stress. The inhibition of plant growth under water stress conditions was intensively proved to be associated with altered plant-water relations. Based on our results, we conclude that the drought exposure imposed evident on root growth as well as plant water-relations. On the other hand, the exogenous application of salicylic acid (SA), trehalose (Tre) or their interaction appeared to mitigate this damage effect of drought with different magnitude throughout counteracting the negative effects of water stress on all growth criteria of root and improving leaf turgidity by decreasing the rate of transpiration, increasing relative water content and decreasing saturation water deficit as well as increasing water use efficiency for economic yield. Grain yield was positively correlated with root fresh mass, root dry mass, root density and root distribution. On the other hand, the grain yield was negatively correlated with root length, root/shoot ratio and the number of adventitious roots of both droughted wheat cultivars. Furthermore, Grain yield positively correlated with transpiration rate, RWC, WUEG, WUEB, osmotic pressure, total soluble sugars, proline, keto acids, citric acid and negatively correlated with SWD, total soluble nitrogen as well as Na+and Cl-.
Keywords: Wheat; Drought; Root growth; Water use efficiency; Transpiration; Relative water content; Saturation water deficit
Abbreviations: RWC: Relative Water Content; SA: Salicylic Acid; SWD: Saturation Water Deficit; Tre: Trehalose; WUE: Water Use Efficiency
Introduction
Stress could lead to marked changes in the growth, morphology and physiology of roots, which would in turn change water and ion uptake; the whole plant is then affected when its root grows in unfavorable medium with less water availability [1]. In this connection, it is well documented that there is marked reduction in root growth rate under water deficit conditions, indicating that this effect is entirely due to the change in plant-water relations [2]. Assessment of root density and distribution could be considered as a key factor for water and nutrient uptake by a plant in soil [3].
Generally, drought is one of the most common stressful conditions that plants experience in their environment, where it has a negative effect on plant-water relations [4]. Transpiration can be regarded as the dominant process in plant-water relations. Many plants limit transpiration by stomatal closure at low negative water potentials originated during the drought period [5]. In this respect, transpiration rate in common bean plants were reported to decrease after four days of withholding water [6]. Similarly, drought induced marked decrease in diurnal and mean daily values of transpiration rate in two wheat cultivars [7].
The pattern of water use is crucial for plants grown with a limited amount of water in soil profile because the plant success depends largely on a sustained water use [7,8]. However, plants are developmentally and physiologically designed by evolution to reduce water use under drought stress [9]. Therefore, the study of water use efficiency (WUE), defined as the ratio of dry matter production to water use, is particularly interesting in situations where growth is affected as a result of limiting water availability [10]. The effect of drought on WUE has been investigated in different plant species such as Zea mays [11].
The inhibition of plant growth under water stress conditions is associated with altered water relations [12]. Hence, less absorbed water means less water content indicated by relative water content (RWC), saturation water deficit (SWD), degree of succulence and degree of sclerophylly [13].
Leaf relative water content (RWC) is proposed as a more important indicator of water status than other water potential parameters under drought conditions, as it is believed that RWC is a reliable parameter for quantifying the plant-drought response [14]. During plant development, water deficiency significantly reduces RWC [15]. A decrease in RWC in response to water deficit had been reported in several studies [16,17]. As a practical proof, Shinde observed that drought considerably reduced RWC in four groundnut varieties. Additionally, waterstressed wheat and rice plants had lower relative water content than non-stressed ones [18].
Yield is a result of the integration of metabolic reactions in plants; consequently any factor that influences this metabolic activity at any period of plant growth can affect the yield [19]. Crop plants are especially sensitive to drought stress during the early reproductive stage, which causes significant yield loss in cereal production and detrimental effects on grain quality. The present study was undertaken to examine the effect of water stress on growth vigor of root as well as osmotic adjustment and solutes allocation in wheat flag leaf of Gemmieza-7 (drought sensitive cultivar) and Sahel-1 (drought tolerant cultivar) during grain filling.
Materials and Methods
Plant material and growth conditions
Pure strains of Triticum aestivum L. Gemmieza-7 (drought sensitive cultivar) and Sahel-1 (drought tolerant cultivar) were kindly supplied by the Agricultural Research Center, Ministry of Agriculture, Giza, Egypt.
For soaking experiment, a homogenous lot of Triticum aestivum L. (i.e. either sensitive or tolerant cultivar) grains were selected. The grains were separately surface sterilized by soaking in 0.01M HgCl2 solution for three minutes, then washed thoroughly with distilled water. The sterilized grains from each cultivar were divided into two sets (≈ 500g per set for each cultivar). Grains of the 1st set were soaked in distilled water to serve as control, while those of the 2nd were soaked in salicylic acid (3mM) for about 6 hours.
After soaking, thoroughly washed grains were drilled in 20 November 2011 and 2012 in plastic pots (20cm in diameter) filled with 5.5kg soil (clay/sand 2/1, v/v), where fifteen grains was sown in each pot. The pots were then kept in a greenhouse at Botany Department, Faculty of Science, Mansoura University, Egypt. The plants were subjected to natural day/ night conditions (minimum/maximum air temperature and relative humidity were 15/25 C and 35/45%; respectively) at mid-day during the experimental period. The plants in all sets were irrigated to field capacity by tap water.
After two weeks from sowing, thinning was started so that five uniform seedlings were left in each pot for the subsequent studies. On the day 65 after planting (at the beginning of heading) the pots of the 1st set was allocated to four groups (20 pots per each group) as follows: control (cont.), water stress (WS), trehalose control, trehalose + water stress (trehalose + WS). The 2nd set group was allocated to four groups as follows: salicylic acid control (SA), salicylic acid + water stress (SA+WS), control trehalose + salicylic acid (SA + trehalose) and salicylic acid + trehalose + water stress (SA+ trehalose +WS). For trehalose (1.5mM) treatment, the plants were sprayed by trehalose 48hrs before starting the stress period and weekly during the stress period.
]
Water deficit was imposed by withholding water at the reproductive stage for 30days within two periods: on the day 65 from planting (heading stage) and at the day 80 from planting (anthesis stage). Each droughted pot received 500ml water at the end of 1st stress period. At the end of stress periods, re-watering to the field capacity was carried out. The undroughted (control) plants were irrigated to the field capacity during the stress period, and all plants were left to grow until grain maturation under normal irrigation with tap water. After thinning and at heading, the plants received 36kg N ha-1 as urea and 25kg P ha-1 as super-phosphate. Samples from flag leaves were taken from each treatment during grainfilling (21 days post-anthesis) (i.e. 106 days after sowing).
Growth vigor of root
To estimate the morphological features of wheat roots grown under different growth conditions, ten replicates from root system of each treatment were harvested. For the root system, root biomass, root length and the number of adventitious roots were estimated.
Monitoring the water status of the soil
Soil water content (SWC) at the end of the stress period was estimated by the destructive method as recommended by Ritchie et al. [20].
Determination of transpiration rate
For measuring transpiration rate, the method of Kanemasu et al. [21] was followed. Before starting transpiration measurement, the soil surfaces in the pots were covered by the projecting margins of the plastic bags lining the pots and wrapping then around the base of stems. This act prevents water loss by direct evaporation from the soil and any measurable loss, hence, represents loss by transpiration. Each pot was weighed periodically during daytime. This was carried out at 3hours intervals, namely at 7am, 10am, 1pm, 4pm and 7pm for one day and the plants were then harvested by cutting just above the soil surface. Fresh weight of leaves was recorded for each pot. Transpiration was expressed in "mg water/g leaf fresh weight/hour". Transpiration rate was calculated for the four daily periods. Actual and saturation vapour pressures (mmHg) at the ambient temperatures were obtained from hygrometric tables and vapour pressure deficit (VPD) was calculated.
Determination of water use efficiency
Water use efficiency was calculated by dividing the grain yield (ton ha-1) or the biomass yield (ton ha-1) by the total amount of water added (gallon). Therefore, water use efficiency for grain yield (WUEG) was calculated from grain yield and water use efficiency for biomass yield (WUEB) was estimated from biomass yield according to Stanhill [22] as following:
WUEG = Grain yield (ton) / Total water used (gallon)
WUEB = Biomass yield (ton) / Total water used (gallon)
Determination of relative water content
For measuring relative water content (RWC), the method of Weatherly [23] and its modification by Weatherly & Barrs [24] was adopted. Leaf discs were punched from the centre of the leaf. They were weighed to estimate their fresh mass (FM), floated for 4 hours on distilled water and weighed again to estimate their turgid mass (TM). For dry mass (DM) determination, the discs were oven-dried at 80ºC till constant weight. Relative water content was calculated as:
RWC = [(FM – DM ) / (TM – DM )] X100
Determination of saturation water deficit
SWD =100 – RWC
Statistical analysis
It should be mentioned that the sample numbers which were taken for investigation were as follows: ten for growth parameters, ten for agronomic traits and three for all chemical analyses and only the mean values were represented in the respective figures and tables. The data were subjected to one-way analysis of variance (ANOVA), and different letters indicate significant differences between treatments at p ≤ 0.05, according to CoHort/ CoStat software, Version 6.311.
Results
As compared to the control values, water stress led to marked decrease (p ≤ 0.05) in root biomass (fresh and dry masses), root density and distribution of both wheat cultivars during grain-filling. On the other hand, water stress induced noticeable increase (p ≤ 0.05) in the root length (nonsignificant increase in case of sensitive cultivar), root/shoot ratio and number of adventitious roots of both wheat cultivars during grain-filling. The magnitude of reduction was greater in drought sensitive cultivar than drought tolerant one. In the majority of cases, application of SA and/or Tre induced a marked increase (p ≤ 0.05) in the values of root biomass (fresh and dry masses), root length (non-significant increase in case of sensitive cultivar) also number of adventitious as well as root/shoot ratio, root density and distribution of both wheat cultivars. However, interaction of SA and Tre was the most effective treatment in increasing all growth vigor of root (Table 1).

Data are represented as means± standard errors from 10 replicates. Different letters within the same column indicate significant differences between treatments at p ≤ 0.05, according to CoHort/ CoStat software, Version 6.311.
Soil moisture content was markedly reduced at the end of the stress period, and it was found to be about 23.3 and 4.6 % (% of oven soil dry weight) for control and droughted pots respectively. Transpiration rate and concurrent effective climatic parameters are shown in Figure 1 & 2. Transpiration rate generally followed the fluctuations in temperature, while it was inversely proportional to relative humidity of air. Transpiration rate in wheat plants and concurrent effective climatic parameters were measured on one day, along with other water relations (relative water content and saturation water deficit). In general, the maximum transpiration rate was attained in the period of mid-day (at 10-1pm) in control and differently treated plants. Water stress caused marked reduction (p ≤ 0.05) in diurnal values of transpiration all over the daytime, and also in the mean daily value of transpiration rate. The application of SA and/or Tre induced significant decrease (p ≤ 0.05) in transpiration rate of water stressed and unstressed wheat plants. Furthermore, the magnitude of decrease in transpiration rate depends mainly on the daytime and on the chemical used. It was clear that grain pre-soaking in SA was more effective than the foliar application of Tre in decreasing the transpiration rate. Moreover, SA and Tre interaction treatment was the more effective treatment than SA or Tre alone especially in the water-stressed plants.


It is obvious from the results in Figure 3 that the values of water use efficiency for grain (WUEG) and for biomass (WUEB) in the stressed plants were significantly lower (p ≤ 0.05) than those of control plants. Comparing both cultivars, the highest WUEG and WUEB values were observed in Sahel-1than Gemmieza-7 under water stress conditions. Application of SA and/or Tre clearly improved WUEG and WUEB in both stressed and unstressed wheat plants. In addition, the treatment with SA and Tre recorded the highest WUEG and WUEB values than the other treatments.
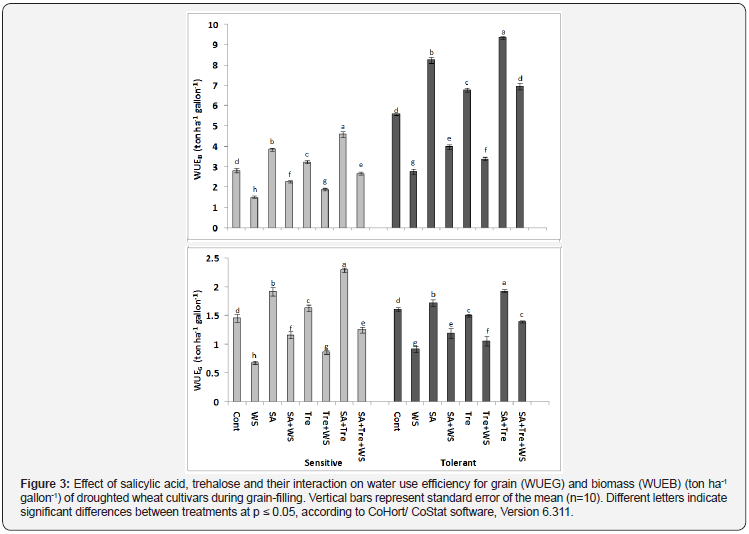
As compared to control plants, water stress caused additional reduction (p ≤ 0.05) in RWC % of wheat flag leaf during grain filling in both cultivars with more reduction in drought sensitive cultivar in comparing to drought tolerant one. Furthermore, the application SA and/or Tre alleviated the deleterious effects of water stress by improving leaf turgidity, where each of these treatments caused significant increase (p ≤ 0.05) in the mean daily values of RWC if compared with stressed plants. This effect was more pronounced with the interaction of SA and Tre interaction (Figure 4 & 5).

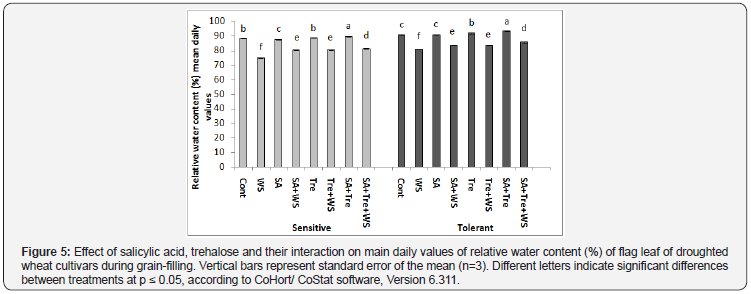


The data in Figure 6 & 7 showed that, water stress increased significantly (p ≤ 0.05) SWD % in flag leaf of both cultivars during grain filling. Comparing both cultivars higher SWD% was observed in drought sensitive cultivar than the drought tolerant one under water stress conditions. Application of SA and/or Tre interaction caused significant decrease (p ≤ 0.05) in SWD as compared with water-stressed plants. SA or SA and Tre interaction was the most effective in decreasing the values of SWD in flag leaf of wheat plants.

The data in Figure 8 revealed that water stress caused significant reduction (p ≤ 0.05) in grain yield of wheat plants. With regard to the wheat cultivar, the sensitive one was more affected by water stress than the tolerant one. SA and/or Tre induced additional increase (p ≤ 0.05) in grain yield of stressed wheat plants. Moreover, the treatment of SA and Tre improved grain yield more than that of SA or Tre only.
Discussion
Plant species and cultivars have different physiological mechanisms in response to drought stress [25]. The use of morpho-physiological and biochemical criteria has been recommended to achieve a rapid and simple screening of highly drought-tolerant individuals. In order to define drought tolerance or sensitivity of both cultivars, growth parameters like lengths, dry and fresh weights of roots were tested under the effect of water-stress during grain-filling. Various environmental stresses that could result in both general and specific effects on their growth and development. Morphological characteristics were shown to be an important factor in controlling the yield of crop plants [26].
The current results showed that drought generally caused a noticeable reduction in almost all growth criteria of root of both wheat cultivars Gemmieza-7 (drought sensitive) and Sahel-1 (drought tolerant) during grain-filling. Extent of reduction was more obvious particularly in Gemmieza-7. These results were in accord with those obtained by Gomaa et al. [27] who postulated that stress-induced inhibition of plant growth as a whole may be due to the elevation in osmotic pressure, which may disturb most cellular physiological and metabolic pathways.
The inhibitory effect of water stress was more pronounced in the sensitive cultivar than in the tolerant one. The variation in response of wheat cultivar for drought stress tolerance was known in many studies [28,29]. In this respect Sankar et al. [30] have reported that the root length, shoot length, total leaf area, fresh weight and dry weight of bhendi are significantly reduced under drought stress treatment. In the present study, water stress greatly decreased root biomass, root density and distribution. On the contrary, root length, number of adventitious roots as well as root to shoot ratio was found to increase as a result of drought stress. In agreement with these results, stressing mulberry plants generally decreased root growth parameters as revealed by Das et al. [31].
Stress could lead to marked changes in the growth, morphology and physiology of roots, which would in turn change water and ion uptake; the whole plant is then affected when its root grows in unfavorable medium with less water availability [1]. In this connection, it is well documented that there is marked reduction in root growth rate under water deficit conditions, indicating that this effect is entirely due to the change in plant-water relations [2].
Morphological characters like fresh and dry masses have a profound effect in water-limited conditions [32]. In the present investigation, both fresh and dry masses of wheat roots were significantly decreased in response to drought. These results appeared to match those obtained by Younis et al. [33] who noticed that root biomass of Vigna sinensis and Zea mays plants was suppressed under stress. Also, Khodary [34] reported that stressing Zea mays plants reduced root fresh and dry masses. The reduction in root biomass can be attributed to the inhibitory effect of stress-induced ABA on cell division and/or cell expansion [35].
The results of the present study also revealed that stress conditions could induce root length and the number of adventitious roots as compared with the unstressed plants . Continuation of root growth under drought stress through stimulation in root length and number of adventitious roots is an adaptive mechanism that facilitates water uptake from deeper soil layers. These results are in accordance with those obtained by Sundaravalli et al. [36] & Yin et al. [37]. Plants must develop vigorous root system that allows them to grow and overcome any stress conditions. Moreover, a large root system may improve a plant’s competitive ability for water during drought stress, which is a plant’s individual survival strategy in natural selection. According to life-history strategy theory, plants with a large root system are partitioning more photosynthetic products to roots, which imply a reduced partition to reproductive growth.
Assessment of root density and distribution could be considered as a key factor for water and nutrient uptake by a plant in soil [3]. In this investigation, stress resulted in significant decrease in both root density and distribution of wheat plants. Root density relates dry mass production to the unit root length, while root distribution represents the fresh mass accumulated per the unit root length.
Stressing wheat plants significantly increased root/shoot ratio when compared with the control plants. This finding coincided with that of many other researchers [13,38]. In this respect, Jabeen et al. [39] suggested that the increase in root/ shoot ratio under water stress conditions may be due to:
(i) The increased accumulation of assimilates diverted to root growth.
(ii) Differential sensitivities of root and shoot to endogenous ABA and/or (iii) greater osmotic adjustment in roots compared with shoots. Matching these assumptions, El-Hendawy [40] assumed that although stress can induce rapid reduction in root growth, shoot growth decreases proportionally more than root growth, causing an increase in the root/shoot ratio.
Drought affects plant water relations, reduces water contents of leaf and plant, causes osmotic stress, inhibits cell expansion and cell division as well as growth of plants as a whole [41]. Determination of water relations is critical for any study of plant resistance to water stress [42]. Plant water status is important not only for plant growth under favorable environmental conditions but also for their ability to tolerate water deficit [43]. Additionally, the importance of the internal water balance in plant water relations is generally accepted because of the close relationship between the balance and turgidity to the rates of physiological processes that control the quality and quantity of growth [44]. Among the common plant-water relations, diurnal changes and mean daily values of relative water content (RWC), saturation water deficit (SWD) and transpiration rate were extensively studied. In addition, water use efficiency (WUE) is of special importance to plant water status particularly under water deficit circumstances. A favorable water balance under water deficit conditions can be achieved either by conserving water, restricting transpiration or by accelerating water uptake.
The results of the present investigation clearly elucidated that stress caused marked reduction in the diurnal and mean daily values of transpiration rate allover the daytime in two wheat cultivars during grains filling. These results were in conformity with those obtained by Trapp et al. [45] pointed out that less water supply could strongly inhibit transpiration.
The decline in transpiration rate in response to water deficit is widely reported in literatures [46] and the effect is more pronounced in susceptible genotypes than in tolerant ones [47].
T he a pplication o f S A a nd/or T re i nduced s ignificant decrease in transpiration rate of water stressed and unstressed wheat plants. Furthermore, the magnitude of decrease in transpiration rate depends mainly on the daytime and on the chemical used. It was clear that grain pre-soaking in SA was more effective than the foliar application of Tre in decreasing the transpiration rate. Moreover, SA and Tre interaction treatment was the more effective treatment than SA or Tre alone especially in the water-stressed plants. These results are in good agreement with those obtained by Aldesuquy et al. [48] studied the effect of antitranspirants (sodium salicylate or ABA) and salinity on some water relations of wheat plants. They found that sodium salicylate or ABA appeared to regain the leaf turgidity of saline-treated plants by inducing additional decreases in transpiration rates and therefore improved the water use efficiency.
Water use efficiency (WUE) is the ratio of dry matter production to water use [10]. Increasing WUE of plants is vital especially to achieve optimum growth with optimal water supply [49]. In this study, droughted wheat plants exhibited lower water use efficiency for grain WUEG and biomass WUEB compared with their unstressed relatives. Comparing both cultivars, higher WUEG and WUEB values were observed in Sahe-1 than Gemmieza-7 under water stress conditions. Water use e fficiency (WUE) has given much attention as a physiological trait related to plant drought resistance. Molecular research regarding WUE enhancement is playing crucial role in selection and cultivation of droughttolerant. When breeding for drought tolerance, biomass productivity and WUE are considered fundamental agronomic characteristics.
Application of SA and/or Tre clearly improved WUEG and WUEB in both stressed and unstressed wheat plants. In addition, the treatment with SA and Tre recorded the highest WUEG and WUEB values than the other treatments.
Leaf relative water content (RWC) has been emphasized as a better indicator of water status of a plant than water potential [39]. The results revealed that drought resulted in noticeable reduction in the mean daily values of RWC with a concomitant increase in SWD of wheat leaves during grain filling in both cultivars. The decrease in RWC is more obvious in drought sensitive cultivar than the drought tolerant one. The suppression of leaf RWC under stress implies that there is a reduction in turgor and that the plant suffers from restricted water availability to the cells [2]. This decrease in turgor under less water availability could conceivably reduce the expansive growth of cells [42]. In this context, marked reduction in RWC of different plant species under water stress conditions was reported in other studies [50,51].
In the present study, the observed reduction in RWC in close parallelism with the increase in SWD. Furthermore, the application SA and/or Tre alleviated the deleterious effects of water stress by increasing leaf turgidity, where each of these treatments caused significant increase in the mean daily values of RWC and significant decrease in the mean daily values of SWD if compared with stressed plants. This effect was more pronounced with the interaction of SA and Tre interaction. These results were similar to those obtained by Aldesuquy et al. [48] using wheat plants grown under salinity conditions and treated with antitranspirants (sodium salicylate or ABA). Also, Kumar et al. [52] reported maintenance of water balance with foliar application of salicylic acid.
Alam et al. [53] reported that combination of Tre with drought showed improved leaf relative water content (RWC) in Brassica species. Similar findings were documented previously by Tre addition with abiotic stress [54,55].
Water stress reduced grain yiled in both wheat cultivars. Crop plants are especially sensitive to drought stress during the early reproductive stage, which causes significant yield loss in cereal production and detrimental effects on grain quality. This is a well established fact that yield of crop plants in drying soil reduces even in tolerant lines of that crop species [32,56]. Drought stress during the early stage of reproductive growth tends to reduce yield by reducing seed number. During seed development stress reduces yield by reducing seed size. Prolonged moisture stress during reproductive growth can severely reduce yield because of reduced seed number and seed size [57]. The yield components, like grain yield, grain number, grain size, and floret number, are decreased under drought stress treatment in sunflower [32]. Moreover, Amin et al. [58] attributed the increase in yield and yield characteristics of onion plants with foliar spray of salicylic acid to an increase in photosynthesis and assimilation and translocation of assimilates, and greater nutrient uptake and increased cytoplasmic streaming and cell integrity. In addition, the promoting effect of SA on the flag leaf blade area and blades area/plant of barley mentioned that enhancing effect of SA on the availability and movement of nutrients could result in stimulating different nutrients in the leaves and consequently promote yield and yield components [59,60].
For growth criteria of root, the grain yield was positively correlated with root fresh mass (r = 0.93, 0.97), root dry mass (r = 0.89,0.95),root density (r = 0.86,0.83)and root distribution(r = 0.93, 0.91). On the other hand, the grain yield was negatively correlated with root length (r = -0.62, -0.39), root/shoot ratio (r = -0.68,-0.56) and the number of adventitious roots (r =-0.66, -0.31) of both droughted wheat cultivars respectively. Furthermore, the grain yield was positively correlated with transpiration rate (r= 0.39, 0.06), RWC (r=0.89, 0.86), WUEG (r= 1.00, 0.94), WUEB (r = 1.00, 1.00), conversely, a negative correlation was recorded for SWD (r = - 0.89, - 0.86).
Conclusion
The applied chemicals appeared to mitigate the negative effect of water stress on growth vigor of root and waterrelations as well as grain yield of both wheat cultivars especially the sensitive one. The effect was more pronounced with SA and Tre treatment. This improvement would result from the promotive effect of the provided chemicals on root growth and recovery of leaf turgidity under water deficit condition. Moreover, key players involved in SA and Tre-induced drought tolerance needs to be clarified using proteomic and genomic approaches.
To Know More About Food and Nutrition
Sciences Open Access Journals Please Click on:
https://juniperpublishers.com/nfsij/index.php
To Know More About Open
Access Journals Publishers Please Click on: Juniper
Publishers
No comments:
Post a Comment